
Optimising the design of a space-based VLBI mission
Ben Hudson
Expansion of VLBI systems into space is crucial for overcoming the inherent limitations of angular resolution defined by the atmosphere’s opacity at sub-mm wavelengths and baseline length of ground-based arrays (practically equal to the Earth’s diameter). Two dedicated space VLBI missions have flown as of the time of writing: VSOP-HALCA and RadioAstron. RadioAstron achieved the finest angular resolution of astronomical observations to date at 7 μas. Numerous concepts for future space-based VLBI systems aiming at even higher resolution, required by various science objectives, have been discussed over the past decade. Amongst the astrophysical challenges which call for these concepts are search and studies of photon rings around black holes and estimates of the latter’s spins, “diving” deeper into the central areas of active galactic nuclei (AGNs) and many others.
TeraHertz Exploration and Zooming-in for Astrophysics (THEZA) is an example of such a concept [1]. Operating on purely space-space baselines, and up to terahertz frequencies, THEZA would provide at least an order of magnitude improvement in resolution compared to the current records set by the Event Horizon Telescope (EHT) on the ground and RadioAstron in space. THEZA would be a highly capable, multiple element space interferometer, with large antennas to achieve sufficient sensitivity to detect photon rings of several orders. This would provide very accurate, astrophysical phenomena-independent tests of strong gravity. An investigation into optimising the orbit configuration to achieve this has been performed, whilst also considering the various engineering challenges [2]. THEZA is a view of the future of space VLBI systems, which would provide access to until now unreachable fields of astrophysics, fundamental physics and cosmology.
Whilst THEZA provides a glimpse of the future of advanced space VLBI, another concept is currently in development by an international team of physicists and engineers. The Black Hole Explorer (BHEX) will observe up to a frequency of ~320 GHz, in collaboration with ground-based arrays [3]. The primary aim of BHEX is to detect the first order photon ring in M87* and Sgr A*. Providing a five-fold increase in angular resolution compared to the EHT, BHEX will also contribute to AGN/jet and black hole demographic studies. BHEX will utilise an antenna of ~3-4m diameter, an optical communications terminal for real-time downlink and will operate in Earth orbit. Figure 1 depicts a simplified, example configuration of BHEX and the preliminary orbit design, able to form ground-space baselines of ~18-33 Gλ, when observing M87*. The (u,v) coverage of M87* and Sgr A* achieved by BHEX in this configuration is shown in Figure 2 (left).
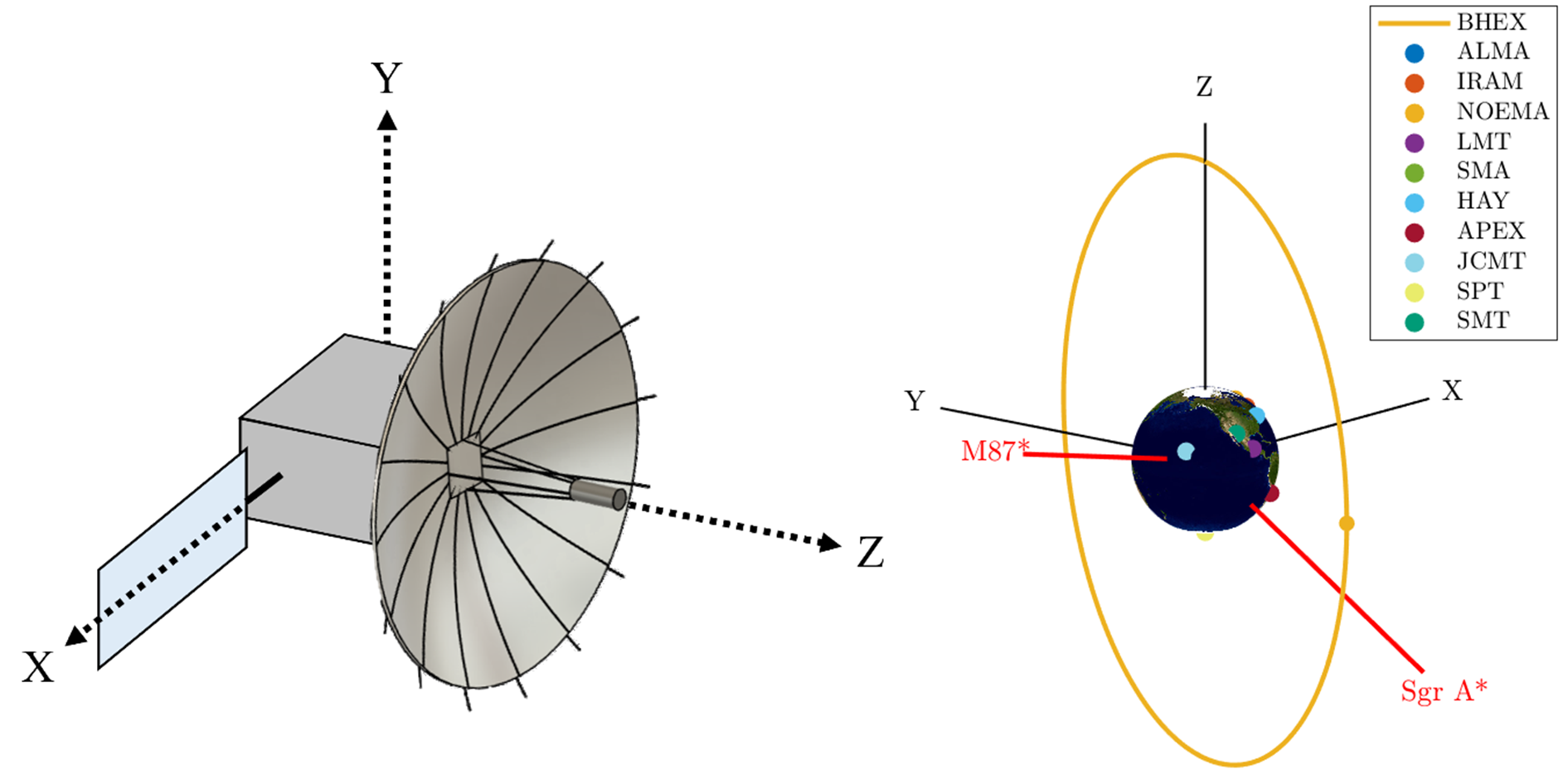
VLBI is a challenging observation technique to perform in space, unique amongst other astronomy methods in the complexity of its operation. Not only does it require a highly performant spacecraft, the mission architecture consists of multiple, complex relationships with systems on the ground (e.g. ground radio telescopes and tracking ground stations). As such, a number of functional constraints, driven by the spacecraft design and mission architecture, can limit when observations can be performed, severely degrading the scientific return of the mission if not considered early in the design process. The functional constraints could include but are not limited to:
- Sun and Earth limb exclusion angles with respect to the antenna pointing (to minimise thermal distortion of the antenna surface)
- Maintenance of a real-time downlink of data during observations
- Radiator surface not pointed towards deep-space, impacting the thermal control of the receivers
For example, BHEX may utilise a real-time downlink with an optical communications terminal. Figure 2 (Right) shows the impact on (u,v) coverage if a particular optical communications terminal configuration is employed. There are positions in the orbit when this link cannot be maintained, and thus observations cannot take place. The reduction in coverage leads to specific changes to the mission’s operations and a complex optimisation problem to maximise observation times for different sources.
Simulating the mission with the ability to model the functional constraints allows these effects to be identified, their impact analysed and mitigation strategies investigated. This is crucial for identifying the key technology requirements and optimising the mission design to provide the maximum science return possible, within financial and programmatic constraints. The coming years will see a focused effort by the BHEX team to move toward a mission implementation in the not too distant future.
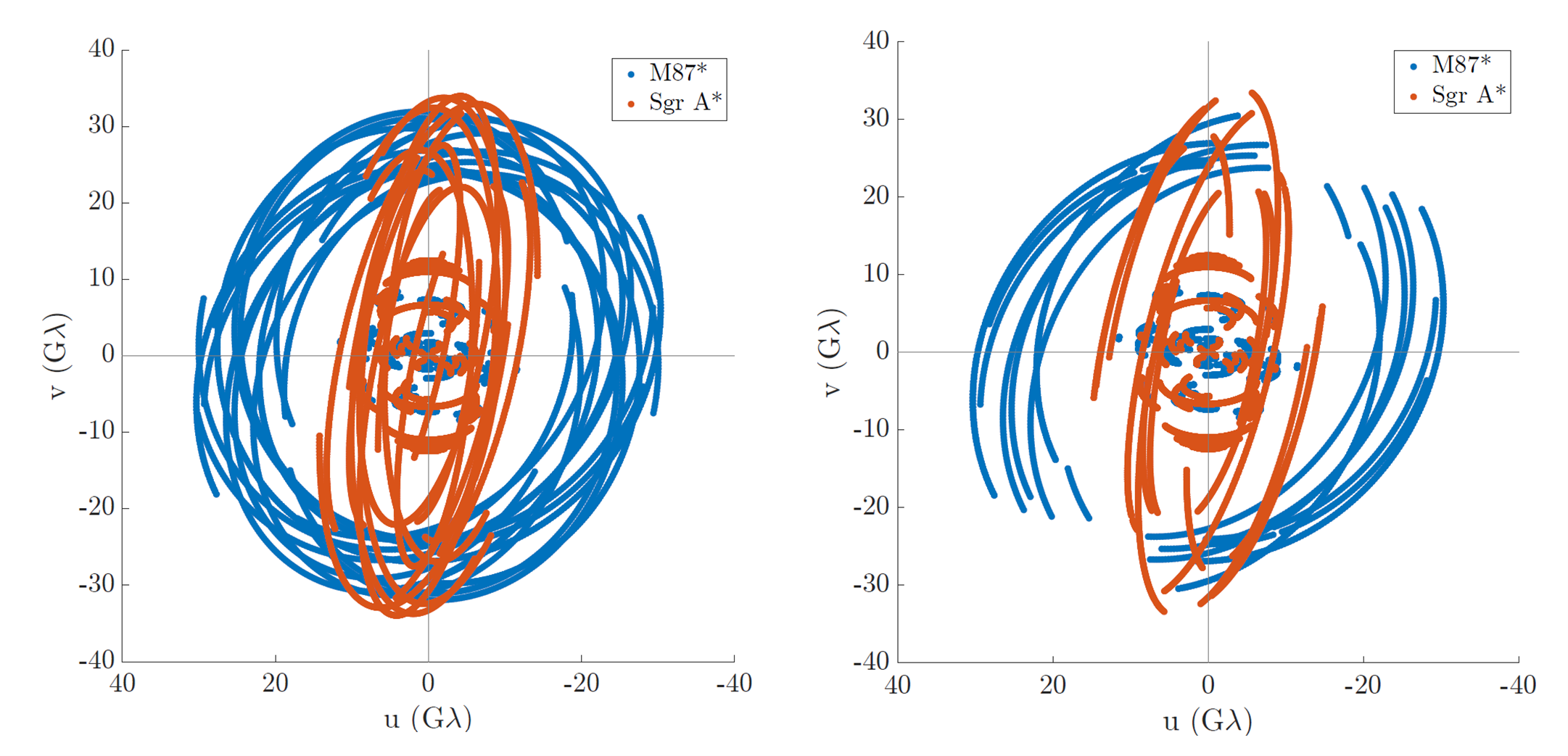
References:
[1] Gurvits, L.I., et al., 2022, The science case and challenges of space-borne sub-millimeter interferometry, Acta Astronautica 196, 314-333.
[2] Hudson, B., Gurvits, L.I, et al., 2023, Orbital configurations of spaceborne interferometers for studying photon rings of supermassive black holes, Acta Astronautica 213, 681-693.
[3] https://www.blackholeexplorer.org/
Contact:
Ben Hudson
Faculty of Aerospace Engineering, Delft University of Technology, Netherlands
KISPE Space Systems Limited, UK
Email: BHudson@kispe.co.uk
Leonid Gurvits
JIVE, Dwingeloo, the Netherlands
Email: lgurvits@jive.eu